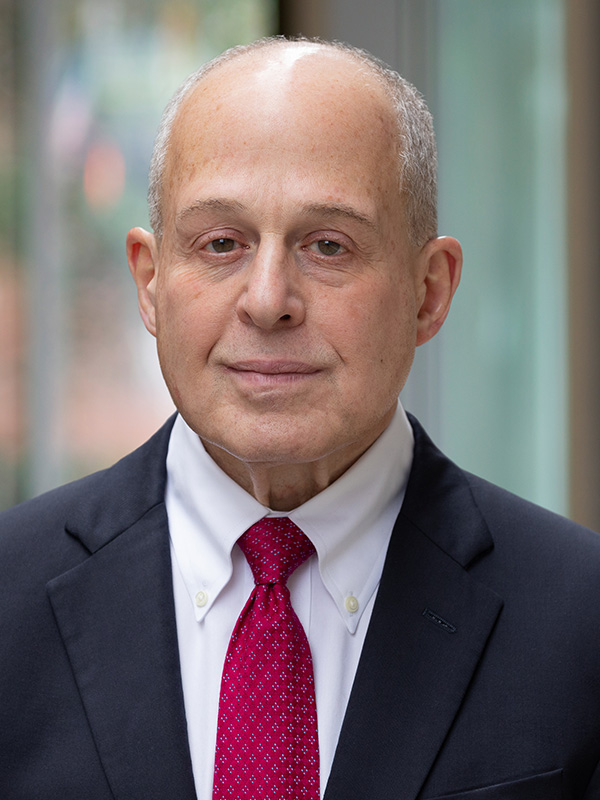
Richard N. Kitsis, M.D.
- Professor, Department of Medicine (Cardiology)
- Professor, Department of Cell Biology
- The Dr. Gerald and Myra Dorros Chair in Cardiovascular Disease
- Director, Wilf Family Cardiovascular Research Institute
Area of research
- Cell Death and Mitochondrial Biology: Fundamental Mechanisms and Roles in Human Disease
Phone
Location
- Albert Einstein College of Medicine Jack and Pearl Resnick Campus 1300 Morris Park Avenue Forchheimer Building G46 Bronx, NY 10461
Research Profiles
Professional Interests
Cell Death and Mitochondrial Biology:
Fundamental Mechanisms and Roles in Human Disease
The most basic decision that any cell makes is to grow (proliferate or hypertrophy), differentiate, or die. Our laboratory studies fundamental mechanisms of cell death and the roles of cell death in normal biology and human disease. At least a dozen cell death programs have been recognized to date (Cell Death Differ, 2018; Physiol Rev, 2019). Little is understood about how they interconnect mechanistically or functionally to bring about cell death. The overarching goal of our work is to create a unified model of cell death that integrates seemingly “individual” cell death programs. Our hypothesis is that these cell death programs, which often undergo activation in the same cell, are components of an integrated cell death response that has arisen over evolution. Thus far, we have elucidated basic aspects of cell death biology including mechanisms that mediate apoptosis and regulated forms of necrosis, molecular interconnections among these programs, and connections with other aspects of mitochondrial biology (Mol Cell, 2004; PNAS, 2007; JBC, 2007; PNAS, 2012; Cell Death Differ, 2014; Nature, 2016; Science, 2018; Nat Commun, 2022; PNAS. 2023; Mol Cell (in press)).
Although our primary focus is basic mechanisms, we are also interested in translational implications. While some of our work has involved models of cancer (Cell Death Differ, 2005; JBC, 2010; Cancer Res, 2011, PLOS One, 2015) and diabetes (Diabetes, 2013; Sci Rep, 2017; Dev Cell, 2021), our primary translational focus is heart disease, the major cause of mortality worldwide. A number of years ago, our lab provided the first evidence that regulated forms of cell death play critical roles in the pathogenesis of myocardial infarction (“heart attack”) (Circulation, 2000; JMCC, 2000; AJP, 2003) and heart failure (JCI, 2003). More recently, the lab has contributed to the development of small molecule and peptide drug prototypes that modulate cell death and may provide the basis for new therapies for heart diseases and other conditions (Nat Chem Biol, 2019; Nat Cancer, 2020). We continue to use cell and in vivo myocardial infarction models in our current work aimed at unifying cell death mechanisms because at least 7 cell death programs are activated in this syndrome.
Current projects
1. A novel platform for caspase-9 activation to bring about necrosis – not apoptosis
We have identified a new molecular platform – distinct from the apoptosome – for caspase-9 activation, which brings about necrosis rather than apoptosis. This pathway appears to operate generally in a variety of cell types as well as in cardiomyocytes during myocardial infarction in vivo. We are currently characterizing the activating complex structurally, activating signals, and downstream pathway (manuscript in preparation).
2. Role of necroptosis in myocardial infarction
Necroptosis, a regulated form of necrosis defined by RIPK3 → MLKL signaling, is important in cardiac damage during myocardial infarction. In many paradigms, RIPK3 is activated by RIPK1. However, we believe that, during myocardial infarction, RIPK3 activation is triggered by the presence of double stranded nucleic acids in the Z-conformation in the cytoplasm. We are currently delineating this pathway, which we suspect may be a prototype for roles of Z-nucleic acids in other aspects of cell signaling. We are also developing a novel small molecule RIPK3 inhibitor as a potential therapeutic in myocardial infarction.
3. Function of the mitochondrial ATP synthase in vivo
The mitochondrial ATP synthase catalyzes the generation of >90% of ATP in eukaryotic cells, but its necessity for maintaining cellular energetics has not been defined in an intact organism. We devised an in vivo strategy to progressively deplete the entire 28-subunit mitochondrial ATP synthase monomer, and dimers thereof, in heart muscle cells, a cell type chosen because of its high energetic demands. Unexpectedly, mice whose heart muscle cells contain 5-15% of the monomeric complex and <2% of dimers remain clinically indistinguishable from controls for weeks, retain normal cardiac function, and exhibit only moderate exercise deficits. Cardiac mitochondrial ATP synthesis rates and concentrations are reduced ~50%, roughly equivalent to those in end-stage human heart failure. Following this period of compensation, all mice transition precipitously to heart failure and die, but remarkably this occurs without further decreases in ATP synthesis rates and ATP concentrations. Experiments are currently underway to understand the compensatory changes that allow mice to cope with such marked depletion of the mitochondrial ATP synthase in an energetically demanding cell type and to delineate the mechanisms that mediate the transition to heart failure.
A second part of this work has been to test a hypothesis that has been advanced by others that the mitochondrial ATP synthase serves a second function as a mitochondrial permeability transition pore, a channel in the inner mitochondrial membrane that mediates necrotic cell death. Our data in isolated mitochondria, cells, and intact mice demonstrate that, rather than serving as the mitochondrial permeability transition pore, the assembled mitochondrial ATP synthase is in fact a negative regulator of this pore (PNAS, 2023).
4. Interrelationships between cellular senescence and cell death in aging
We have performed a genome-wide CRISPR screen to identify proteins that direct cells under stress toward a senescent versus cell death fate. We are currently studying these molecules in models of senescence relevant to aging. The long-term goals are to understand the determinants of senescence versus cell death as cellular reactions to stress and to devise novel senotherapeutics to divert damaged cells from a senescent toward a cell death fate.
5. ER/SR-mitochondrial relationships in regulating necrotic cell death and metabolism
We have previously created novel peptides and small molecules that conformationally active or inhibit mitofusins (MFNs) (Nature, 2016; Science, 2018), which are large GTPases involved in mitochondrial fusion and ER/SR-mitochondrial tethering. We are now using these reagents to perturb the physical distance and interactions between ER/SR and mitochondria. We have shown that MFN inhibitors limit infarct size during myocardial ischemia/reperfusion even when dosed at the time of reperfusion (manuscript in preparation). We are also attempting to do the opposite: augment ER-mitochondrial interactions and Ca2+ transfer to boost myocardial metabolism during heart failure.
6. Regulation of ferroptosis by OPA1
We have discovered that OPA1 markedly sensitizes cells to ferroptosis, an iron-dependent form of necrotic cell death mediated by hydroperoxidation of phospholipids in cellular membranes. Unexpectedly, this sensitization to ferroptosis is independent of the ability of OPA1 to promote mitochondrial fusion. Rather, by maintaining mitochondrial homeostasis and function, OPA1 both stimulates the generation of mitochondrial lipid ROS and suppresses an ATF4-mediated integrated stress response that would otherwise upregulate mechanisms that inhibit ferroptosis (Molecular Cell, accepted). This axis has potential implications for several diseases.
Selected Publications
Link to publications:
https://pubmed.ncbi.nlm.nih.gov/?term=kitsis+rn&sort=date
Link to current NIH funding:
https://reporter.nih.gov/search/evTFsgrZrkiK9bKr9tpWVA/projects?projects=Active