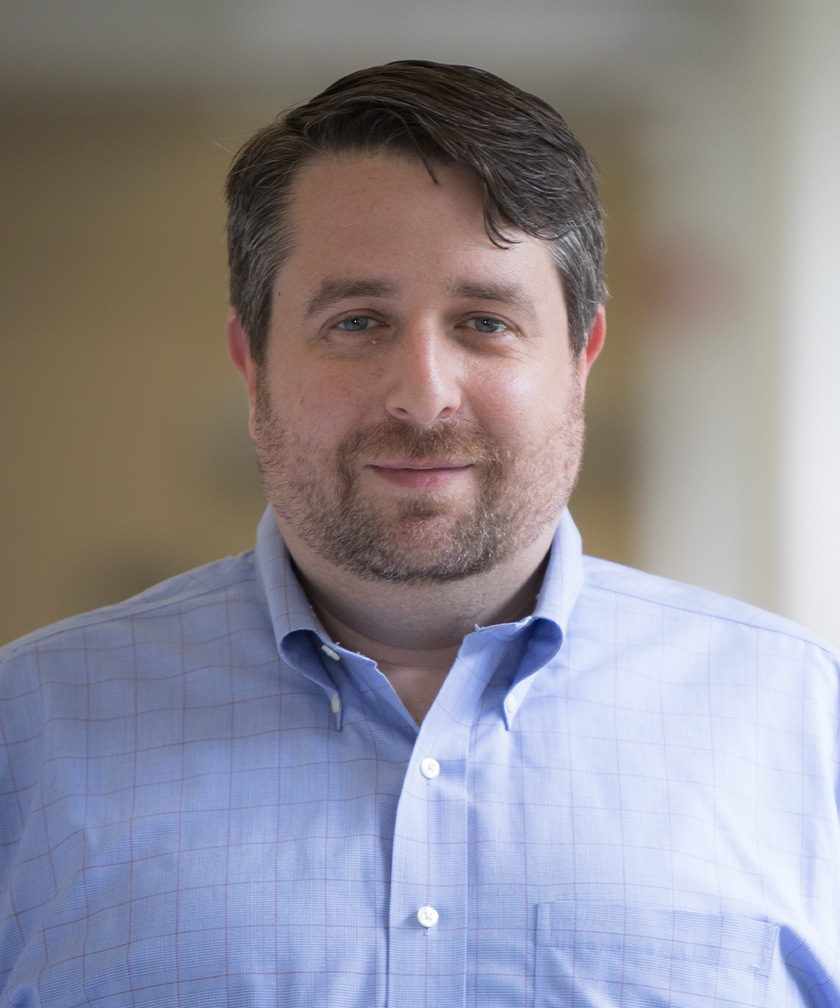
Matthew J. Gamble, Ph.D.
- Professor, Department of Molecular Pharmacology
- Professor, Department of Cell Biology
Area of research
- Histone variant macroH2A; Cancer; Cellular senescence; Chromatin structure and function; Transcription; Splicing; Senescence-associated secretory phenotype; Endoplasmic reticulum stress responses; Poly(ADP-ribose) polymerase
Phone
Location
- Albert Einstein College of Medicine Jack and Pearl Resnick Campus 1300 Morris Park Avenue Golding Building 202 Bronx, NY 10461
Research Profiles
Professional Interests
MacroH2As, histone variants with diverse roles in gene expression and DNA damage responses – The macroH2A-type histone variants (which include macroH2A1.1, macroH2A1.2 and macroH2A2) have roles in tumor suppression, cellular senescence, activation and repression of transcription, promotion of DNA repair and suppression of the reprogramming of differentiated cells into stem cells. MacroH2As are typified by a histone H2A-like region fused by a flexible linker to a C-terminal macrodomain, a ligand-binding domains whose functions is modulated by binding to poly(ADP-ribose) produced by a family of poly(ADP-ribose) polymerases. MacroH2A1 regulates the expression of genes found within its large chromatin domains which can span hundreds of kilobases. Through changes in its expression and/or alterations in its genomic localization, disruption of macroH2A1’s tumor suppressive functions are common in cancer; alterations of macroH2A transcription and splicing occur in a variety of cancers including those of lung, breast, colon, ovaries, endometrium, bladder, testicles, and melanocytes. Consistently, macroH2A1 loss in primary cells is sufficient to trigger an oncogenic gene expression profile. We are interested in many aspects of macroH2A biology. 1) How are macroH2As targeted to specific regions of the genome? 2) How does macroH2A1.1 in collaboration with PARPs regulate gene expression? 3) How does macroH2A1 regulate chromatin accessibility at enhancers? 4) How does macroH2A participate in DNA repair? 5) What regulates macroH2A1’s alternative splicing?
Chromatin dynamics during oncogene-induced senescence and cancer – Oncogene-induced senescence (OIS) is an important tumor suppressive mechanism whereby a cell harboring an oncogenic mutation enters a stable proliferative arrest. At the same time the senescent cell secretes a host of inflammatory cytokines, chemokines and metalloprotease called the senescence-associated secretory phenotype (SASP), which serves to recruit immune cells to clear the senescent cells from tissues. The histone variant macroH2A1 plays a critical role in the transcriptional regulation of SASP genes during senescence. We are currently studying the mechanism by which macroH2A regulates the SASP response. We hypothesize that changes in macroH2A1 expression, seen in many cancers, allows these cells to bypass senescence and proceed on the pathway towards transformation.
Interplay between transcriptional elongation rates and alternative splicing – Alternative splicing is a crucial aspect of gene expression, allowing a gene to yield functionally distinct products, the abundance of which are regulated by cellular cues. Splicing dysregulation is central to several cancers and developmental diseases. Alternative splicing can be regulated through the recruitment of splicing factors which promote or repress distinct splicing events. Splicing largely occurs co-transcriptionally, and so, splicing outcomes are also affected by aspects of the transcription process and chromatin environment. The local elongation rate of RNA polymerase II is one aspect of transcription with important consequences on splicing outcomes. A barrier to progress in the field has been the lack of a high-throughput assay to measure splicing rates in mammalian cells. To address this, we have developed SKaTER-seq (Splicing Kinetics and Transcript Elongation Rates through sequencing). With this assay, we are exploring a myriad of factors that regulate splicing, including elongation rate, gene architecture, binding sites for RNA binding factors, chromatin structure and histone modifications. With this powerful approach we will determine the underlying causes of spicing alterations in disease.
Selected Publications
Kim, J., Sun, C., Tran, A.D., Chin, P.J., Ruiz, P.D., Wang, K., Gibbons, R.J., Gamble, M.J., Liu, Y. and Oberdeorffer, P. (2019) "The macroH2A1.2 histone variant links ATRX loss to alternative telomere lengthening." Nat. Struct. Mol. Biol. 26:213-219.
Ruiz, P.D. and Gamble, M.J. (2018) "MacroH2A1 chromatin specification requires its docking domain and acetylation of H2B lysine 20." Nat. Commun. 9:5143.
Hodge, D.Q., Cui, J., Gamble, M.J. and Guo, W. (2018) "Histone variant macroH2A1 plays an isoform-specific role in suppressing epithelial-mesenchynmal transition." Sci. Rep. 8:841.
Chen, H., Ruiz, P.D., McKimpson, W.M., Novikov, L., Kitsis, R.N. and Gamble, M.J. (2015) “MacroH2A1 and ATM play opposing roles in paracrine senescence and the senescence-associated secretory phenotype.†Mol. Cell 59:719-31.
Chen, H., Ruiz, P.D., Novikov, L., Casill, A.D., Park, J.W. and Gamble, M.J. (2014) “MacroH2A1.1 and PARP-1 cooperate to regulate transcription by promoting CBP-mediated H2B acetylation.†Nat. Struct. Mol. Biol. 21:981-9.
Hussey, K.M., Chen, H., Yang, C., Park, E., Hah, N., Erdjument-Bromage, H., Tempst, P., Gamble, M.J.*, Kraus, W.L.* (2014) “The histone variant macroH2A1 regulates target gene expression in part by recruiting the transcriptional coregulator PELP1.†Mol Cell Biol. 34:2437-49.
Gamble, M.J. (2013) Expanding the functional repertoire of macrodomains. Nat Struct Mol Biol 20:407-8
Zhang, T., Berrocal, J.G., Yao, J., DuMond, M.E., Krishnakumar, R., Ruhl, D.D., Gamble, M.J., and Kraus, W.L. (2012) “Regulation of poly(ADP-ribose) polymerase-1-dependent gene expression through promoter-directed recruitment of a nuclear NAD+ synthase.†J. Biol. Chem. 287:12405-16.
Novikov, L., Klerman, H., Jalloh, A.S., and Gamble, M.J. (2011) “QKI-mediated alternative splicing of the histone variant macroH2A1 regulates cancer cell proliferation.†Mol Cell Biol. 31:4244-4255
Zhang, X., Gamble M.J., Stadler, S., Cherrington, B.D., Causey, C.P., Thompson, P.R., Robertson, M.S., Kraus, W.L., Coonrod, S.A. (2011) “Genome-wide analysis reveals PADI4 Cooperates with ELK-1 to activate c-Fos expression in Breast Cancer Cells.†PLoS Genetics. 7:e1002112.
Gamble, M.J. and Kraus, W.L. (2010) “Multiple facets of the unique histone variant macroH2A: from genomics to cell biology.†Cell Cycle 9:2568-2574.
Gamble, M.J., Frizzell, K.M., Yang, C., Krishnakumar, R., Kraus, W.L. (2010) “The histone variant macroH2A1 marks repressed autosomal chromatin, but protects a subset of its target genes from silencing.†Genes and Development 24:21-32
Frizzell, K.M., Gamble, M.J., Zhang, T., Berrocal, J.G., Zhang, T., Krishnakumar, R., Cen, Y., Sauve, A.A., and Kraus, W.L. (2009) "Global Analysis of Transcriptional Regulation by Poly(ADP-ribose) Polymerase-1 and Poly(ADP-ribose) Glycohydrolase in MCF-7 Human Breast Cancer Cells." Molecular and Cellular Biology. 284:33926-28
Zhang, T., Berrocal, J.G., Frizzell, K.M., Gamble, M.J., Dumond, M.E., Krishnakumar, R., Yang, T., Sauve, A.A., Kraus, W.L. (2009) “Enzymes in the NAD+ Salvage Pathway Regulate SIRT1 Activity at Target Gene Promoters.†J. Biol Chem. 284:20408-17
Krishnakumar, R.*, Gamble, M.J.*,Frizzell, K.M., Berrocal, J.G., Kininis, M., Kraus, W.L. (2008) Reciprocal binding of PARP-1 and histone H1 at promoters specifies transcriptional outcomes. Science 319:819-21 (* equal contribution)
Gamble, M.J. and Fisher R.P. (2007) SET and PARP1 remove DEK from chromatin to permit access by the transcription machinery. Nat Struct Mol Biol. 14;548-55
Gamble, M.J. and Kraus W.L. (2007) Visualizing the histone code on LSD1. Cell 128:433-4
Larochelle, S., Batliner, J., Gamble, M.J., Barboza, N.M., Kraybill, B.C., Blethrow, J.D., Shokat, K.M., Fisher, R.P.(2006) Dichotomous but stringent substrate selection by the dual-function Cdk7 complex revealed by chemical genetics. Nat Struct Mol Biol 13:55-62
Gamble, M.J., Erdjument-Bromage, H., Tempst, P., Freedman, L.P., Fisher, R.P. (2005) The histone chaperone, TAF-I/SET, is required for activated transcription in vitro of chromatin templates. Mol Cell Biol 25:797-807
Gamble, M.J., Freedman, L.P. (2002) A coactivator code for transcription. Trends Biochem Sci 27: 165-7.
Rachez, C., Gamble, M.J., Chang, C.P., Atkins, G.B., Lazar, M.A. Freedman, L.P. (2000) The DRIP complex and SRC-1/p160 coactivators share similar nuclear receptor binding determinants but constitute functionally distinct complexes. Mol Cell Biol 20:2718-26.
Rachez, C., Lemon, BD., Suldan, Z., Bromleigh, V., Gamble, M., Naar, A.M., Erdjument-Bromage, H., Tempst, P., and Freedman, L.P. (1999) Ligand-dependent transcription activation by nuclear receptors requires the DRIP complex. Nature 398:824-8.