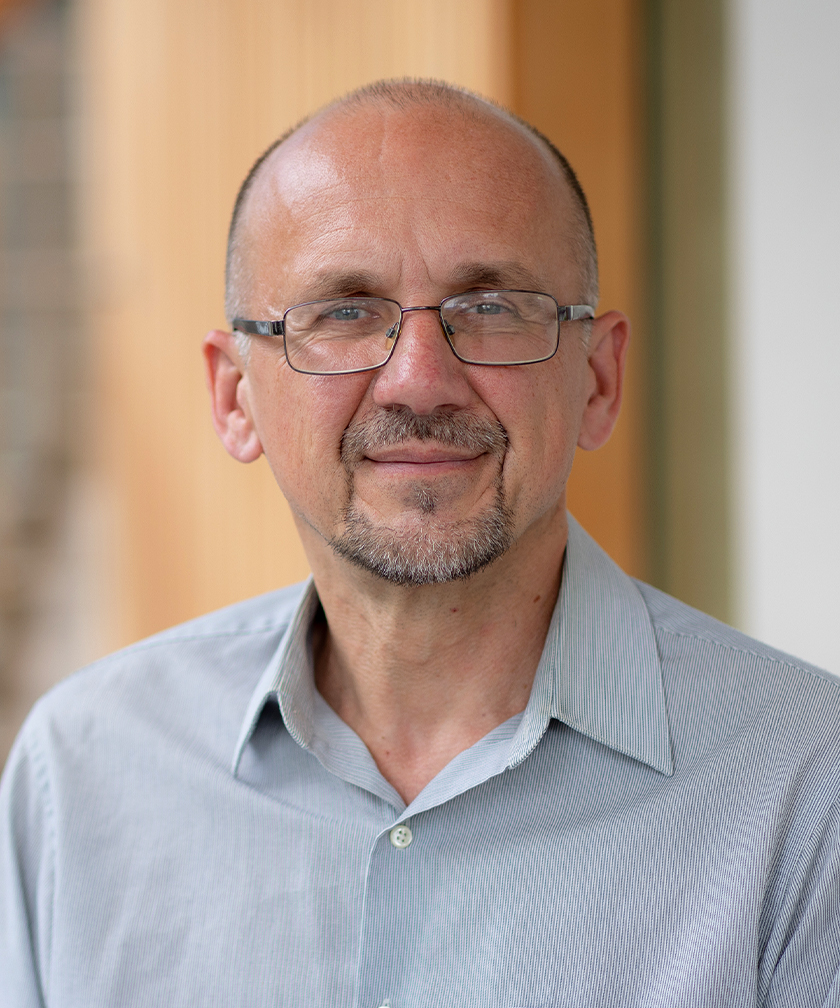
Vladislav Verkhusha, Ph.D.
- Professor, Department of Genetics
Area of research
- Engineering of fluorescent proteins and biosensors for super-resolution microscopy and deep-tissue imaging. Designing of genetically encoded optogenetic tools for manipulation of biological processes with light in vivo.
Phone
Location
- Albert Einstein College of Medicine Jack and Pearl Resnick Campus 1300 Morris Park Avenue Ullmann Building 1217 Bronx, NY 10461
Research Profiles
Professional Interests
Non-invasive optical imaging, monitoring and manipulation of metabolic processes in living mammals is more feasible within the near-infrared (NIR) optical transparency window (650-900 nm) where hemoglobin and melanin absorbance significantly decreases, and water absorbance is still low. The most red-shifted fluorescent proteins (FPs) of the GFP-like family have excitation and emission spectra outside of the NIR region and suffer from low brightness and modest photostability. Natural bacterial phytochrome photoreceptors (BphPs) utilize an enzymatic product of heme, low-molecular-weight biliverdin, as a chromophore.
BphPs provide many advantages over other natural chromophore-containing proteins. Unlike the chromophores of non-bacterial phytochromes, biliverdin is ubiquitous in mammals. This makes BphP applications in mammalian cells, tissues and whole mammals as easy as conventional GFP-like FPs, without supplying chromophore through an external solution. BphPs exhibit NIR absorbance and fluorescence, which are red-shifted relative to that of any other phytochromes, and lie within the NIR optical window. This makes BphPs spectrally complementary to other existing optical probes and optogenetic tools based on the GFP, flavoprotein and rhodopsin-like protein families. Independent domain architecture and pronounced conformational changes upon biliverdin photoisomerization make BphPs attractive templates to design various photocontrollable genetically-encoded probes.
In our laboratory, we engineer new BphP-based FPs, biosensors and optogenetic tools. These include bright and spectrally resolvable permanently fluorescent NIR FPs, photoactivatable with non-phototoxic NIR light FPs, and reversibly photoswitchable FPs. We also focus on designing NIR reporters for protein interactions and biosensors for intracellular ions and metabolites. Lastly, we engineer BphPs into optogenetic elements allowing us to noninvasively regulate intracellular processes in vivo with NIR light.
We apply various directed protein evolution approaches based on rational structure-based design and random mutagenesis of template BphPs, high-throughput flow cytometry and multiwell plate spectroscopy. These conventional techniques allow screening for standard properties of genetically encoded probes, such as excitation and emission wavelengths, brightness, photostability, pH stability and folding efficiency. We also develop new protein engineering and high-throughput approaches to specifically optimize BphP-based constructs. These include time-resolved fluorescence lifetime measurements, expression in bacterial periplasmic space, screening of mutant libraries in yeast and in mammalian cells using shuttle vectors and inducible somatic hypermutations.
The resulting NIR probes, biosensors and molecular tools are tested in mouse models and applied to various in vivo studies. These NIR constructs extend optical methods to multicolor deep-tissue in vivo imaging, cell and tissue labeling, photoactivation and tracking, and detection of enzymatic activities and protein interactions in cells, tissues and whole mammals. The engineered NIR optogenetic tools allow light-manipulations of cellular processes directly through the skin of living animals.
Selected Publications
Leopold A.V., Thankachan S., Yang C., Gerashchenko D., and Verkhusha V.V. A general approach for engineering RTKs optically controlled with far-red light. Nature Methods 2022, 19: published online Jun 9. doi:10.1038/s41592-022-01517-z.
Oliinyk O.S., Baloban M., Clark C.L., Carey E., Pletnev S., Nimmerjahn A., and Verkhusha V.V. Single-domain near-infrared protein provides a scaffold for antigen-dependent fluorescent nanobodies. Nature Methods 2022, 19: 740-750.
Kasatkina L.A., Ma C., Matlashov M.E., Vu T., Li M., Kaberniuk A.A., Yao J., and Verkhusha V.V. Optogenetic manipulation and photoacoustic imaging using a near-infrared transgenic mouse model. Nature Communications 2022, 13: 2813.
Shemetov A.A., Monakhov M.V., Zhang Q., Canton-Josh J.E., Kumar M., Chen M., Matlashov M.M., Li R., Yang W., Nie L., Shcherbakova D.M., Kozorovitskiy Ye., Yao J., Ji N., and Verkhusha V.V. A near-infrared genetically encoded calcium indicator for in vivo imaging. Nature Biotechnology 2021, 39: 368-377.
Kaberniuk A.A., Baloban M., Monakhov M.V., Shcherbakova D.M. and Verkhusha V.V. Single-component near-infrared optogenetic systems for gene transcription regulation. Nature Communications 2021, 12: 3859.
Manoilov K.Y., Verkhusha V.V., and Shcherbakova D.M. A guide to the optogenetic regulation of endogenous molecules. Nature Methods 2021, 18: 1027–1037.
Redchuk T.A., Karasev M.M., Donnelly S.K., Hülsemann M., Virtanen J., Moore H.M., Vartiainen M.K., Hodgson L. and Verkhusha V.V. Optogenetic regulation of endogenous proteins. Nature Communications 2020, 11: 605.
Matlashov M.E., Shcherbakova D.M., Alvelid J., Baloban M., Pennacchietti F., Shemetov A.A., Testa I. and Verkhusha V.V. A set of monomeric near-infrared fluorescent proteins for multicolor imaging across scales. Nature Communications 2020, 11: 239.
Leopold A.V., Chernov K.G., Shemetov A.A. and Verkhusha V.V. Neurotrophin receptor tyrosine kinases regulated with near-infrared light. Nature Communications 2019, 10: 1129.
Yang J., Li L., Shemetov A.A., Lee S., Zhao Y., Liu Y., Shen Y., Li J., Oka Y., Verkhusha V.V., and Wang L.V. Focusing light inside live tissue using reversibly switchable bacterial phytochrome as a genetically encoded photochromic guide star. Science Advances 2019, 5: eaay1211.
Oliinyk O.S., Shemetov A.A., Pletnev S., Shcherbakova D.M. and Verkhusha V.V. Smallest near-infrared fluorescent protein evolved from cyanobacteriochrome as a versatile tag for spectral multiplexing. Nature Communications 2019, 10: 279.
Shcherbakova D.M., Cammer N.C., Huisman T.M., Verkhusha V.V. and Hodgson L. Direct multiplex imaging and optogenetics of Rho GTPases enabled by near-infrared FRET. Nature Chemical Biology 2018, 14: 591-600.
Li L., Shemetov A.A., Baloban M., Hu P., Zhu L., Shcherbakova D.M., Zhang R., Shi J., Yao J., Wang L.V. and Verkhusha V.V. Small near-infrared photochromic protein for photoacoustic multi-contrast imaging and detection of protein interactions in vivo. Nature Communications 2018, 9: 2734.
Leopold A.V., Chernov K.G., and Verkhusha V.V. Optogenetically controlled protein kinases for regulation of cellular signaling. Chemical Society Reviews 2018, 47: 2454-2484.
Pennacchietti F., Serebrovskaya E.O., Faro A.R., Lukyanov K.A., Verkhusha V.V., Mishin A.S., Testa I. Fast reversibly photoswitching red fluorescent proteins for live-cell RESOLFT nanoscopy. Nature Methods 2018, 15: 601-604.
Redchuk T.A., Kaberniuk A.A. and Verkhusha V.V. Near-infrared light-controlled systems for gene transcription regulation, protein targeting and spectral multiplexing. Nature Protocols 2018, 13: 1121-1136.
Redchuk T.A., Omelina E.S., Chernov K.G., and Verkhusha V.V. Near-infrared optogenetic pair for protein regulation and spectral multiplexing. Nature Chemical Biology 2017, 13: 633-639.
Chernov K.G., Redchuk T.A., Omelina E.S., Verkhusha V.V. Near-infrared fluorescent proteins, biosensors and optogenetic tools engineered from phytochromes. Chemical Reviews 2017, 117: 6423-6446.
Kaberniuk A.A., Shemetov A.A. and Verkhusha V.V. A bacterial phytochrome-based optogenetic system controllable with near-infrared light. Nature Methods 2016, 13: 591-597.
Shcherbakova D.M., Baloban M., Emelyanov A.V., Brenowitz M., Guo P. and Verkhusha V.V. Bright monomeric near-infrared fluorescent proteins as tags and biosensors for multiscale imaging. Nature Communications 2016, 7: 12405.
Yao J., Kaberniuk A.A., Li L., Shcherbakova D.M., Zhang R., Wang L., Li G., Verkhusha V.V. and Wang L.V. Multiscale photoacoustic tomography using reversibly switchable bacterial phytochrome as a near-infrared photochromic probe. Nature Methods 2016, 13: 67-73.